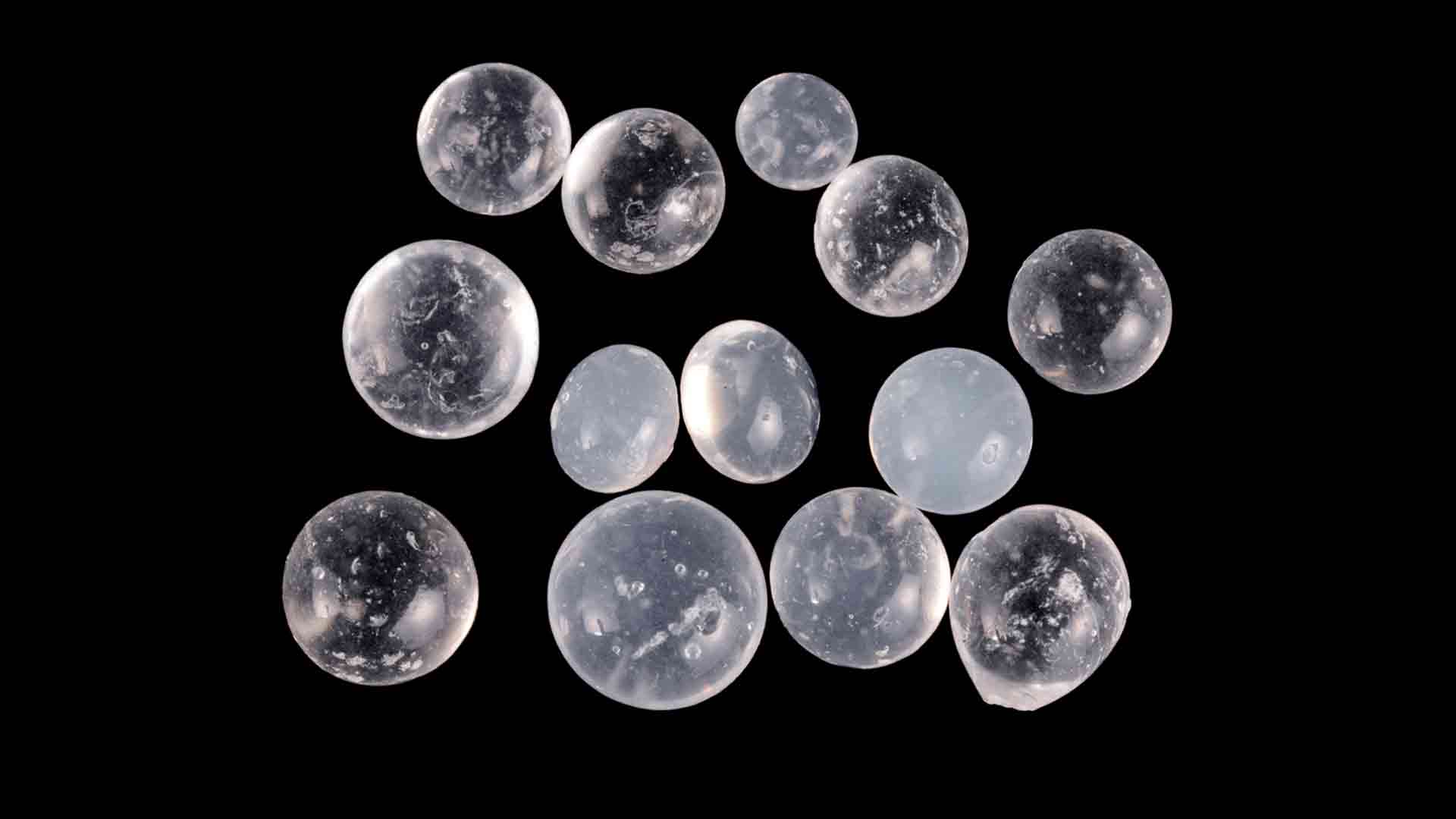
Porous and ultra-lightweight, aerogels are nanostructured materials synthesized from a gel where the liquid component is replaced with a gas. The first aerogel developed from silica was reported by Kistler in 1931. By the 1990s, NASA was using them for thermal insulation in spacecraft, space suits, and blankets. Since then, aerogels have been adopted for insulation in subsea systems, oil refineries, industrial pipelines, buildings, refrigerators, and clothing like jackets and shoe inserts.
Novel drying methods for these materials result in the formation of a robust, ultra-lightweight, dendritic microstructure consisting of pores smaller than 100 nm and 90 to 99.8% of empty space. Since these pores are too small for air to travel through, aerogels are highly effective insulators.
Due to innovations in processing these materials, aerogels are now being developed with many properties, making them potentially useful in applications beyond insulation, such as energy storage, catalysis, drug delivery, and more. Recent advancements have led to more durable options with studies focused on enhancing their structural integrity and thermal properties. Aerogels also exhibit a high specific surface area for a non-powder material, a low mean free path for diffusion, low thermal conductivity, low acoustic velocity, low refractive index, low dielectric constant, and extremely low density (ranging from 0.0011 to ~0.5 g/cm³).
We examined the CAS Content CollectionTM, the largest human-curated collection of scientific information, to find out where these breakthroughs in materials science are having the greatest impact.
Are aerogels “frozen smoke?”
Some of the earliest aerogels developed — silica aerogels — were known as “frozen smoke” because of their lightweight, ultra-low-density properties. However, while all aerogels are extremely light, they aren’t made of smoke as their nickname suggests.
There are three main types of aerogels based on their composition: inorganic aerogels, organic aerogels, and aerogel composites. Inorganic aerogels not only encompass silica aerogels but also metal oxide aerogels and chalcogenide aerogels, which are typically synthesized from inorganic precursor materials like metal alkoxides or metal salts. Organic aerogels comprise carbon aerogels, carbon nanotube aerogels, graphene aerogels, and polymeric aerogels, all of which are synthesized from organic precursor materials like phenol formaldehyde resin. Composite aerogels include mixed-oxide aerogels, aerogel-metal organic framework (MOF) composites, aerogel-Mxene composites, and aerogels synthesized by combining inorganic and organic precursors.
Our analysis of the CAS Content Collection shows the extensive growth in publications relating to aerogels over the last two decades. Since 2013, the number of patents has risen consistently, even more than journals in some years, indicating significant commercial interest in the field (see Figure 1).
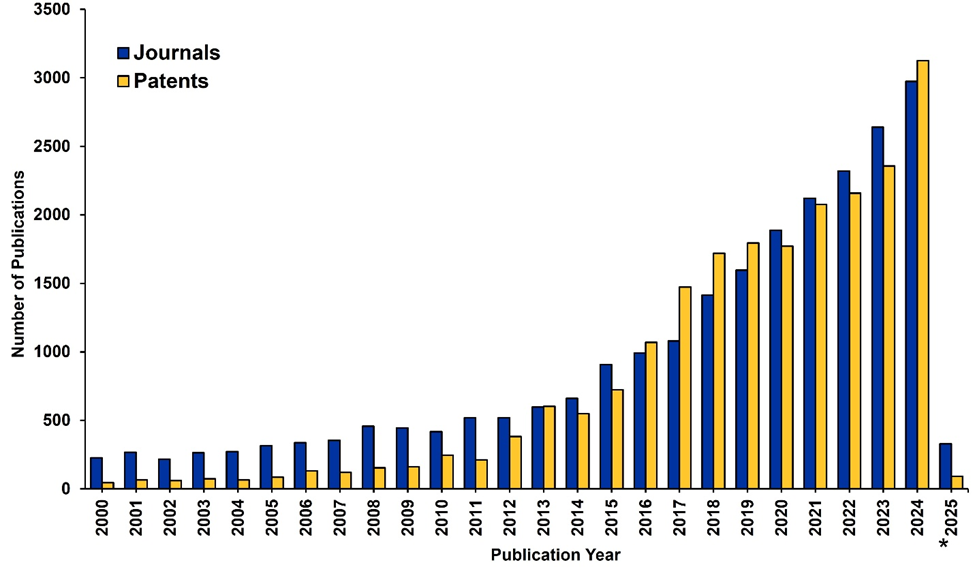
For certain types of aerogels, patents have even surpassed journals — inorganic aerogels that have been in the market for many years, for example, as well as newer synthetic polymer aerogels (see Figure 2). The newest group, aerogel composites, understandably has fewer related publications, and journals are slightly outpacing patent publications so far.
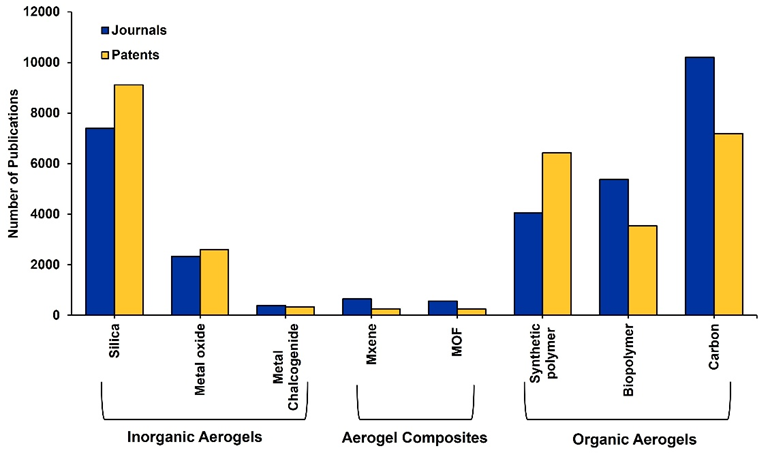
We also analyzed the top 20 indexed substances within the CAS Content Collection™ from journal publications and patents (Figure 3), which correlate closely with the document volume presented in Figure 2. These substances are the most used materials for a particular class of aerogel synthesis.

Exploring different types of aerogels
The aerogel market is anticipated to experience a compound annual growth rate (CAGR) of approximately 17% throughout the forecast period of 2025-2035. What are these different aerogels being developed, and how might they be used?
Polymeric aerogels: The properties of synthetic polymer aerogels closely resemble those of silica-based aerogels, and their enhanced insulating and mechanical strength makes them suitable for applications in aerospace, energy storage and conversion, and protective equipment such as bulletproof vest, knee pads, and sports equipment. The development of biodegradable and bio-based polymers is also gaining momentum, as these materials present a sustainable alternative for aerogel materials. Their potential for functionalization endows them with specific properties suitable for biomedical applications like tissue engineering, regenerative medicine, and drug delivery systems.
Inorganic aerogels: Metal oxide aerogels exhibit exceptional thermo-chemical stability due to the inherent characteristics of their inorganic ion crystal structures, making them suitable for catalytic applications. Similarly, chalcogenide aerogels are composed primarily of high-quality semiconductor materials, making them promising for advanced applications like sensors, LEDs, photovoltaics, photocatalysis, and electrocatalysis.
Composite aerogels: Mxene and MOF-based aerogel composites are recent developments and remain in the research phase. Notably, the strategic integration of 2D Mxene sheets into a 3D porous aerogel exhibits outstanding electrical conductivity, mechanical robustness, and high specific capacitance outperforming the conventional supercapacitor electrode materials. Their flexibility and lightweight nature also make them ideal for portable and flexible electronics.
The synthesis of MOF-based aerogels has also revolutionized materials science by integrating the micro/mesoporosity of MOFs with the meso/macroporosity of aerogels, yielding structures with exceptional morphological, mechanical, and physicochemical properties. These advanced materials have already found numerous applications as supercapacitors and in processes including catalysis, drug delivery, sensing, energy storage, water treatment, and environmental remediation.
Unique applications of aerogels
It’s clear that aerogels show considerable promise in energy storage, catalysis, and various biomedical applications. It is also noteworthy that the cosmetics and acoustics (soundproofing) industries have more patent publications than journals, highlighting the commercialization of aerogels in these fields (see Figure 4).
With their ability to diminish the speed and amplitude of sound waves, aerogels can be used as acoustic insulation materials. In cosmetics, aerogels function as anti-shine agents in creams. Composite aerogels of TiO2-silica are being added to sunscreen because of their photoprotective properties, which can increase SPF beyond what conventional formulations can achieve. These uses demonstrate the potential of aerogels to improve all types of products, including consumer goods.
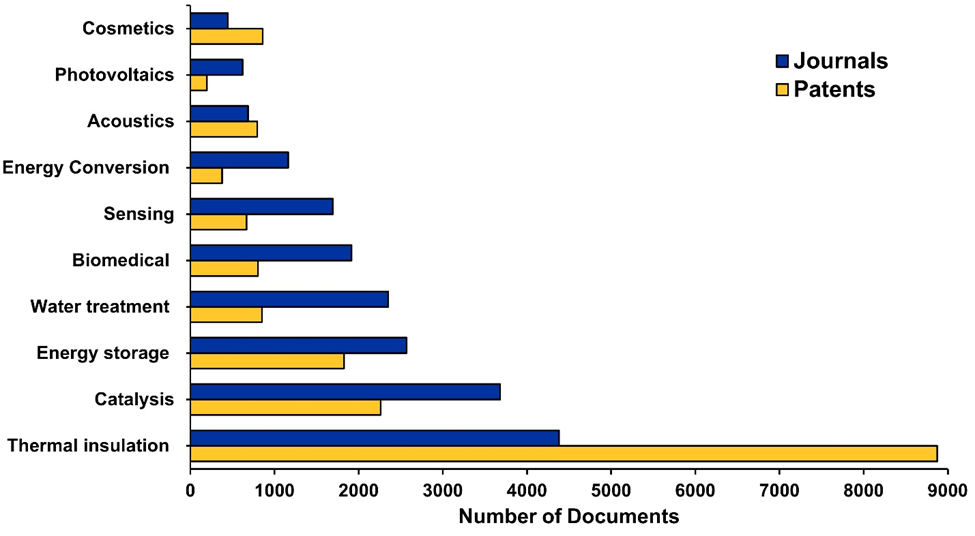
Overcoming challenges to commercialization
Despite many recent advances, aerogels still have drawbacks such as low mechanical strength and fragile texture that can limit their applications. The development of composite and polymer aerogels is addressing these issues, but more work remains to bring them into wider usage.
Their production remains an intricate, time-consuming process, and while laboratory-scale production has been achieved, scaling up to industrial-level production without compromising the quality and properties presents a substantial obstacle. It’s also crucial that researchers find ways to reduce the production costs associated with aerogels — a related issue to the problem of scale.
The aerogels market is still in its early stages, so it’s no surprise that certain challenges remain. However, the future for these materials is bright. As scientists continue to harness their full potential, aerogels will play more impressive roles in applications from energy to medicine and more.