The mRNA vaccine success story
Messenger RNA (mRNA) vaccines are a familiar concept to many, owing to their role in substantially altering the course of the COVID-19 pandemic and preventing millions of deaths. However, they are not a novel discovery. In fact, the therapeutic potential of mRNA can be linked back to the 1980s when it was hypothesized that mRNA could be used as a drug when delivered to a target via lipid droplets. Since then, mRNA vaccines have been designed to target an array of pathogens, including zika, rabies, influenza, and cytomegalovirus. Figure 1 below outlines the mechanism of action of mRNA vaccines for inducing cell- and antibody-mediated immunity.
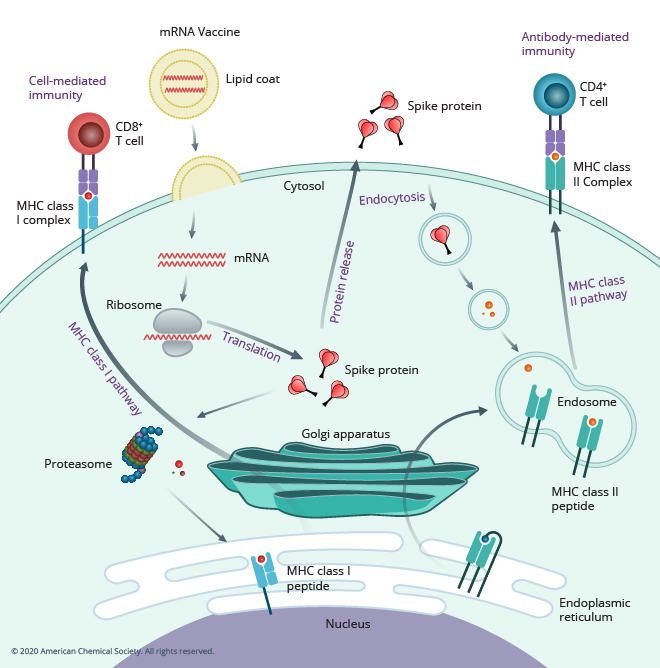
In contrast to conventional vaccine approaches, which directly introduce antigenic proteins that stimulate an immune response in the host, mRNA vaccines introduce mRNA encoding to a disease-specific antigen and leverage the host cells’ protein synthesis machinery to produce antigens that elicit an immune response. The production of these foreign antigens within the body prepares the immune system to recognize and memorize this viral antigen so it is ready to fight off future infections caused by a virus with the same antigen.
Watch this video to see how an mRNA vaccine uses our bodies’ cells to generate immunity to COVID-19.
mRNA vaccine: the long and winding road
The successful application of mRNA vaccine technology to combat COVID-19 would not have been possible without the pioneering work of biochemists, immunologists, and developmental biologists. But the road to success has been long and winding, with decades of dead ends and disputes over technology. Researchers initially found mRNA technology a struggle to work with due to its instability: a challenge that was largely overcome with the development of lipid nanoparticles (LNPs). Encapsulating mRNAs within these protective little fat bubbles enables them to be shuttled to the right place in cells without degrading.
While initial studies into mRNA vaccines looked promising, the cost of optimizing and upscaling vaccine platforms was a major limiting factor to large-scale rollout. Early attempts to develop and commercialize mRNA vaccines were abandoned due to manufacturing challenges, including a vaccine for avian influenza. Many of the candidate vaccines never progressed to in-human studies, and companies such as Shire and Novartis sold their mRNA vaccine portfolios. Companies could not see the economic potential in the technology.
Rise of the COVID-19 mRNA vaccine
The COVID-19 pandemic had a big impact on vaccine development. Suddenly, mRNA was rapidly and successfully deployed as a vaccine to treat the novel coronavirus, SARS-CoV-2. Through a coordinated research effort, two mRNA vaccine candidates were quickly awarded emergency approval to fight COVID-19. These vaccines offered several advantages over conventional vaccines, including:
- Increased specificity and efficacy resulting from induction of both B- and T-cell immune responses.
- Ease of production in large quantities in a cell‐free environment by in vitro transcription (IVT), which allows for faster development, a simplified production process, and more cost-effective manufacturing
- Safety advantages, namely the absence of integration into the host cell genome and no DNA interaction (thus, no mutational risk to the host), no viral particle formation, and transient antigen expression (limiting its persistence in the body).
The concerted efforts of scientists around the globe during the COVID-19 pandemic has accelerated mRNA vaccine development and helped us to overcome the challenges that hindered early research. The knowledge garnered from the pandemic will be valuable to the field of vaccine technology and the quest in producing future vaccine design using RNA approaches.
The mRNA vaccine pipeline
Bolstered by the success of the COVID-19 mRNA vaccines, around 90 lead developers are developing mRNA vaccine candidates for a vast array of pathogens. Moderna alone is developing mRNA vaccines to combat Epstein-Barr virus, cytomegalovirus, seasonal flu, and respiratory syncytial virus. Plans to develop mRNA vaccines for Herpes simplex virus, multiple sclerosis, cancer, and human immunodeficiency virus are also in the works. Clinical trials on the first mRNA-based malaria vaccine are set to start this year, with the hopes of tackling this long-neglected disease. The applications of this technology are seemingly limitless.
A glimpse into the pipeline shows that researchers are exploring a range of mRNA technology formats, including modified, non-modified, and self-amplifying mRNAs. While LNP formulation remains the most popular approach for delivering the mRNA to its target, alternative delivery vehicles, such as cationic nano-emulsions, and polymers, are also being explored. Developers believe that these new formulations may bring advantages in stability, potency, immunogenicity, and valency. However, with approximately three-quarters of mRNA vaccine candidates in the preclinical/exploratory phase of development, it will be several years before we see how these new technologies fare in clinical trials.
Optimizing mRNA vaccines for future use
Though the field of mRNA vaccines has advanced in recent years, several process development challenges remain, such as Plasmid DNA supply, the complexity of in vitro transcription and encapsulation processes, varying mRNA impurity profiles, and the need for ultra-cold storage.
There are other factors that reinforce the need for continued innovation, such as the risk of potential emergence of viral variants (as seen with COVID-19) and the need for high dose administration and subsequent injection site reactions in individuals being vaccinated against SARS-CoV-2.
Stability
Despite being an important attribute, minimal research has been performed investigating the stability profile of mRNA drug products, e.g., LNP-mRNA and protein mRNA complexes. include several that investigate the effects of freeze-drying on mRNA integrity. Other approaches include spray-drying mRNA and generating LyoSpheres (freeze-dried droplets with mRNA). This area of research will be crucial for large-scale mRNA vaccine deployment in the future.
Cost
As aforementioned, cost was a key limitation to the advancement of mRNA vaccines in the early days, and this is set to remain an important consideration. Currently, relatively high amounts of RNA are required to produce a vaccine, which not only costs time and money, but also increases the likelihood of potential side effects (more on this shortly). Furthermore, the ultra-low temperature storage of -70°C is costly, requiring special freezers that may not be normally present at distribution or vaccination centers. Researchers predict that investments in the manufacturing infrastructure and raw materials required for mRNA vaccines will also lower the cost of these vaccines in due time.
Lowering the dose
One way to navigate the challenges of RNA dose lowering is by using self-amplifying RNA.
It is similar to RNA in terms of structure, but much larger, encoding a replicase that enables amplification of the original stand of RNA upon delivery into the cell. The result is a much higher yield of protein requiring a minimal dose of RNA, leading to additional cost and efficiency benefits. However, one potential issue is the size of the molecule and the impact of this on delivery.
mRNA vaccines have been in use for years, but their clinical potential remained untapped until the advent of a global pandemic. Substantial progress has been made in the space of a few years. The priorities are clear in terms of what’s required to produce a new generation of mRNA vaccines. Watch this space for new developments.
A therapeutic world beyond mRNA vaccines
To uncover the world of RNA-derived therapeutics beyond mRNA vaccines, see our insights report “RNA-Derived Medicines: A review of the research trends and developments”, on the application of RNA in medicine and how chemical modifications and nanotechnology can enhance the delivery and efficacy of RNA pharmaceuticals.